Color is a universal language. At traffic lights around the world, drivers understand to stop on red and go on green. For many signaling applications, including those in the railroad and aerospace industry, operators are trained to recognize and respond to very specific colors. So how do you make sure that your light fixture outputs the right color of light to meet specification?
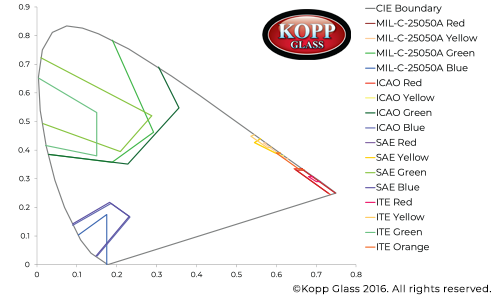
The color of light emitted from a light fixture can be described by its chromaticity, which is a three-coordinate value. Lighting fixtures that have the same chromaticity values will output identically colored light. Often, global lighting standards for chromaticity are used to ensure reliability, consistency, and safety in aerospace and transportation applications. These include specifications from the International Civil Aviation Organization (ICAO), the Federal Aviation Administration (FAA), the Institute of Transportation Engineers (ITE), the Association of American Railroads (AAR), and the Defense Logistics Agency (Mil) among others.
Three factors will determine the chromaticity of a light fixture: the light source, the transmission of the lens or cover material, and the human eye. While you can’t change the way that color is perceived by the eye, you can adjust the light source and the lens material to achieve a specific chromaticity output.
3 Factors That Determine Chromaticity
Light Source
Every light source has a unique spectral power distribution that influences the color that a light fixture produces. A light source can be broadband, emitting widely across the visible spectrum, or discrete, emitting only within narrow wavelength ranges. Many fixtures use a white light source, such as an incandescent bulb or a white LED, in combination with a colored cover lens to achieve target chromaticity values. You can also produce specific colors by using a colored LED with either a clear or colored lens.
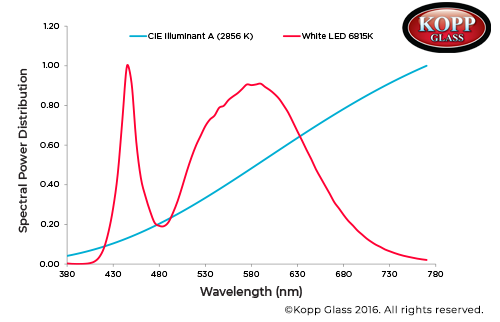
The light source that you choose for your fixture will influence the chromaticity of the light output. This is because of differences in spectral power distributions, as shown in the image to the right. Every wavelength of visible light emitted from a source contributes to the appearance of color. A white LED, with a narrow peak in the blue wavelengths from the LED semi-conductor chip and a broader phosphor generated peak from green to red wavelengths, will not contribute to chromaticity in the same way as an incandescent light source, which has an irradiance that increases with longer wavelengths over the entire visible light range.
As LED adoption increases, it’s important to remember that LEDs are not a direct replacement for incandescent bulbs. This is especially true when you need to achieve a specific chromaticity output. When changing light sources, you must take the spectral power distributions of your old and new light sources into consideration. Typically, adjustments will need to be made to the glass composition of the lens to achieve your required chromaticity, which we discuss in the next section.
Glass Color and Light Absorption
Like the light source of your fixture, the material that you choose for your lens will impact the chromaticity of the light output. Different materials will absorb different wavelengths of light, and each wavelength of visible light that can pass through the lens will contribute to its color. A blue glass, with the transmission spectrum shown in the figure below, transmits mainly blue wavelengths of light while absorbing strongly in the yellow, orange, and red regions. Depending on the specific light source you chose, your light output color could range from blue to blueish-green.
Every material-especially glasses-has a unique transmission spectrum, which is determined by its composition. Glasses can be ionically colored, where transition metal or rare-earth ions in the glass network selectively absorb wavelengths of light. These glasses, referred to as bandpass filters, are often characterized by broad absorption bands that produce blue, amethyst, and green colors. Glasses can also be colored with a combination of small nano-crystals and a thermal striking process that creates glasses used to absorb light with shorter wavelengths. These glasses have sharp-cut absorption edges that yield red, yellow, and orange colors. The transmission spectra for various colors of glass are shown in the image below.
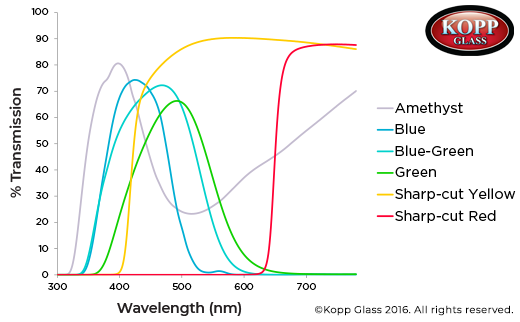
But are all blues the same? When choosing between different materials of similar color, such as glass and plastic, there is no guarantee that two ‘blue’ lenses will produce the same color of light. They may look the same to the naked eye, but they could produce different colors when illuminated. To ensure the light output of your fixture meets chromaticity requirements, you need to consider the transmission spectrum of each material and how it pairs with your light source.
Unlike colored coatings, which absorb or reflect light predominantly at the surface of the material, glasses are intrinsically colored. Accordingly, the thickness of a glass lens will influence its chromaticity value; thicker lenses absorb more light and produce darker, more saturated colors. In addition, the color of a glass is permanent. Its transmission spectrum and chromaticity will remain constant even after prolonged exposure to heat, ultraviolet (UV) light, and other environmental factors.
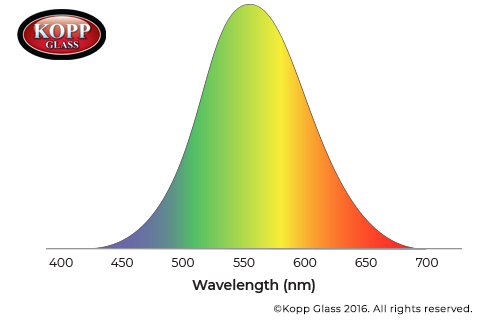
Eye Sensitivity
The perception of color by the human eye is the one thing that an engineer has no control over. Color perception begins when light hits the retina, which contains photoreceptors called rods and cones. Rods are more sensitive and are responsible for our low-light vision; their peak sensitivity is at 500nm. Cones are less sensitive and are responsible for our daylight and color vision. There are three types of cones: L cones have peak sensitivities at red wavelengths, centered around 560 nm, M cones have peak sensitivities at green wavelengths, centered around 530 nm, and S cones have peak sensitivities at blue wavelengths, centered around 420 nm. As light hits these photoreceptors, a nerve impulse is sent to the brain, which interprets this light according to its color.
Calculating Chromaticity Within Different Color Spaces
Chromaticity is a three-coordinate value that is mapped onto a color space, where light spectra are numerically described according to the stimulus of the eyes by light. This is done by the use of three color-matching functions that correspond to the peak wavelengths of the photoreceptor cones.
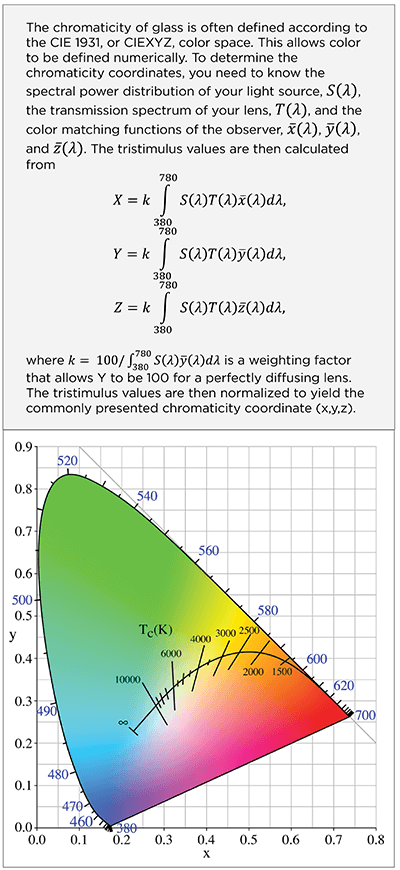
The spectral power distribution of the light output from a fixture is weighted by each of the three color-matching functions and then integrated over visible light wavelengths. The result is three tristimulus values that give an objective description of the color. Within the CIE 1931 color space, these values are X, Y, and Z. These values are then normalized to give the chromaticity of the light, (x,y, z).
The CIE 1931 color space, mapped in the image to the right, is particularly informative, since the tristimulus values describe red (x), green (y), and blue (z) wavelengths of light, and the Y value gives an indication of the brightness or intensity of the light output.
Other color spaces include CIE 1964 and CIELAB, CIELUV, RGB, and HSB. It should be noted that most color spaces can be converted between one another through the use of mathematical expressions. However, most signal lighting specifications use either CIE 1931 or CIE 1964 chromaticity values.
Designing to Meet Chromaticity Specifications
LED adoption is accelerating; they offer numerous benefits that are hard to ignore. As their performance improves and costs decrease, many lighting applications are switching to LEDs. But as shown above, when a light source is changed, there are no guarantees that the chromaticity will be the same-or remain within specification. Furthermore, the specifications you need to meet may be different as well. This is true of airport runway and obstruction lighting; if a fixture uses an incandescent bulb, it must meet SAE-AS25050A color requirements. However, when using a light source other than an incandescent or xenon bulb, the requirements will be outlined in Engineering Brief 67D. We'll examine the differences between these two colors spaces in more depth in an upcoming article.
The lighting industry is rapidly evolving; in this fast-paced, competitive environment, it’s critical to embrace new technologies, such as LEDs. Otherwise, you run the risk of obsolescence. But before making heavy investments into LED technology, their impact needs to be fully understood. You must evaluate all parts of the equation-source, lens, and specifications-before you make the switch. You may need to change your lens, or even adhere to different specifications for varying light sources. To make the transition as simple and informed as possible, work with your suppliers. You’ll be able to reap the benefits that LEDs provide while staying within specification.